Developing Winners: Narrowing the field of CO2 Reduction Technologies
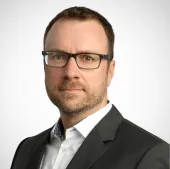
By Dr. Andrew J. Stewart, Vice President, Cement Manufacturing & Supply Chain, Eastern Canada
In order to secure a more livable and sustainable future, the world is decarbonizing and Lafarge Canada has a role to play in this. Over the past couple of years, the startup world has been at the door of emissions-intensive industries—which is both unusual and invigorating. There are many entrepreneurs, scores of ideas, and in some cases, quite a bit of capital working on a CO₂ solution for emitters such as cement plants. Several dozen companies have sought to help Lafarge Canada decarbonize in the recent past, but unfortunately, a strong solution at a global scale, which is economically reasonable, remains an enigma—although locally, several directions have been showing great promise.
Due to the large number of ideas—some well thought out and others more dubious—Lafarge Canada has had to work towards a framework to evaluate options rapidly and identify the high-potential solutions to avoid spending too many resources on the less viable initiatives. This framework is highly beneficial not only to industrial peers but also to those with new ideas, with the ultimate goal of helping facilitate efficiency.
To enable filtering through the herd, Lafarge Canada’s team started by asking a few fundamental questions to decide how much interest and effort to put into these new ideas, and even whether to bring them into the project or partnership pipelines.
Is it scalable?
The scale of CO₂ emissions is immense. For example, a cement plant could have 1 million tonnes per year (tpy) of CO₂ or more to address. Those experienced in heavy industry have a feel for things measured in tonnes and thousands of tonnes, but new participants often struggle to comprehend the magnitude beyond ‘a lot.’ What does 1 million tonnes look like? How about 5 million? It is often hard to physically comprehend these amounts—for example, 5 million tonnes of gravel would nearly fill Wembley Stadium, but would not build even a modest mountain.
This question often raises a red flag for solutions that use the captured CO₂ to generate a new product. The new product could incorporate anywhere between a few percent to 60% CO₂ or more, but the usefulness or economics of "that much" of something is often very poor. Market value for specialty products of a few thousand tonnes does not transfer when a few million tonnes of the same thing is made, yet the economics is still calculated. Even before knowing if chemically or engineering-wise it would actually work, a mass balance should be made, and determined: is this a CO₂ solution that can grow to scale?
Are the resources available to make it happen?
A large proportion of solutions proposed to address the issue of CO₂ emissions either bind the CO₂ to something else to make a new material or stabilize the CO₂ somehow for the longer term. Critical in this style of pathway is that the "something" that the CO₂ is bound to is available at scale (or could be), which means at least tens of thousands of tonnes at a single location. Materials of lesser availability may contribute and could be economically favourable, but as they will not solve the issue broadly, the economics must be strong.
As Lafarge Canada reviews the resources, it has become a recurring theme to take a look at the electrical and water requirements for these various technologies as well. Often, the magnitude demanded for those two specific limited resources is staggering. It has become not uncommon to see processes so intensive that a relatively modest CO₂ abatement system could require the full generation of a large hydroelectric dam and the entire flow of a fairly substantial freshwater river. Hence, it is worth considering these points before becoming too enthusiastic about an idea.
Does it technically work and is it permanent?
It seems painfully obvious, but there are numerous ideas moving quite far forward, sometimes even at lightning speed, that make little technical sense. Technological Readiness Levels (TRLs) have become more popular to reference lately and are helping to frame the maturity of ideas. Nevertheless, the framework is self-policed, and there is a tendency for exaggeration of TRL level, for example with proven equipment technologies that are being applied to novel uses (e.g. “the equipment is available and off the shelf – TRL Level 10!”), even though the actual application might be much lower.
Another worthwhile note comes back to the quote from the American writer, Upton Sinclair: “It is difficult to get a man to understand something when his salary depends on his not understanding it.” Conscious or not, startups need to reach the next funding round and the sprint can blind them to fundamental issues. Chemistry, engineering, and business intersect in this space, so there is a lot of grey zone to enjoy. It is recommended to be diligent and skeptical, nevertheless with an optimistic outlook towards new ideas; at early stages, be transparent about concerns as to where the big hurdles will be to begin addressing them early on.
The other question here is if the technical solution presented has the potential for permanency. While purported to be CO₂ capture solutions, many systems are indeed of benefit but really focus on the reduction or reuse of CO₂ rather than permanent abatement. For instance, sustainable aviation fuel, SAF, is a system that captures CO₂ from emitters and converts it into aircraft fuel. There is a clear benefit to this process as the fossil fuel emissions from traditional fuels are avoided as essentially the carbon molecules are used twice and emitted once (used once in the industrial process, then captured and used again for SAF, then emitted into the atmosphere). This is an improvement over direct emission from the industrial sector, however, as an isolated system (ignoring positive additional developments like biogenic CO₂ for the moment), it offers a 50% CO₂ reduction rather than the 100% aimed for – nevertheless a massive step.
Do logistics impede viability?
Another potential trap to fall into is ignoring logistics. High volume and relatively low value supply chain costs tend to be dominated by logistics. Business cases that do not include the cost of moving materials or CO₂ to come together in one place and then moving the new material to its final destination have overseen a large piece of the cost. A new, classic case of such a paradigm is distant undersea CO₂ sequestration – transportation infrastructure and operational costs can dominate the overall system. Another example would be alternative natural resources that could be employed but are geographically remote.
Is it financially viable?
To create a sustainable CO₂ solution within the industry, there needs to finally be a financial payback to proceed or a reasonable pathway to reach one. Either CO₂ emission costs or the foregoing of positive incentives for reductions will generally be the next best alternative of a “do-nothing” scenario depending on the regulatory system in the particular jurisdiction. While there has been a broader commitment across industries aiming for NetZero 2050, financially negative payback investments will not enable businesses to move rapidly past subsidised demonstration projects. The capital intensity is simply too high to sustain negative return projects mid-term. Threading the loop between lowering CO₂ footprint and doing so in an economically viable way is the central key of the current sustainability challenge.
With the answers to the screening questions above, a successful route can be defined and, in many cases, a “kill-early” approach is best to avoid wasting time. Even topics which may not be widely scalable may have strong positives in other regards and can be extremely suitable as a local, and profitable, partial solution – this framework can help clarify those as well.
Is it too difficult?
This is a question that is important, but not one to address too early on. All of the answers are difficult; the hard-to-abate industries are capital-demanding, necessitating efficient processes and a high momentum. Rather than framing the next consideration in the view of how hard it will be, it is perhaps better to consider how long it will take. If a likely outcome for a specific model is 15 – 20 years away, it is not ripe for “implementers” to spend time on it today. However, if it looks 10 years out, the question becomes how can it be supported to be 5 years away?
An example
To conclude, provided below is an example of how these questions would be screened. A new start-up “CO₂ – No Do” has developed a novel idea to capture blast furnace emission CO₂ and convert it into polycarbonate greenhouse windows.
- Is it scalable?
Polycarbonate is widely used in the global market (estimated at about 5 million tonnes), which is a big advantage. Polycarbonates are made up of repeating chains of carbonate groups, C₁₅H₁₆O₂, hence for one piece of the chain, 15 carbon atoms are needed, meaning 15 CO₂ molecules per polycarbonate chain piece. To consider the chemistry, for every gram of polycarbonate, 2.4g of CO₂ would be needed (it is higher as the oxygen would be released). Therefore, with a significant emission source of 1 million tonnes of CO₂, a polycarbonate solution would generate about 400,000 tonnes of polycarbonate – about 8% of the global market. At this stage, it can already be concluded that this technology has the potential to be a large abatement method, although maybe not at full industry scale. Where the right conditions exist, it could be a full-site solution, but it is unlikely to be an industry-wide solution as the volumes of polycarbonate become too high for the available outlets. - Are the resources available to make it happen?
As polycarbonate is made of carbon, oxygen, and hydrogen, the elements are extremely common and would be available globally in significant amounts. Other materials needed for the process would need to be reviewed, but the basics are there to not be limited by physical resources. No details on electrical or water demands are available at this stage, so this would be probed in the near future to better understand the process requirements. - Does it technically work and is it permanent?
In this case, it would be assumed that the process technology works and inputs, other than the CO₂, are CO₂-free. As polycarbonate would have an application, near-term the material would be a CO₂ store, long term depending on the waste market of a particular geography, polycarbonate could be reused, recycled, or used as a fuel back into an industrial process (and then CO₂ captured). As such, there is good potential for a reasonably permanent solution here. - Do logistics impede viability?
With the wide usage of polycarbonate and industrial emitters tending to be near population centers, at first glance, the idea would pass this hurdle. - Is it financially viable?
A quick search for polycarbonate pricing shows about US$2.50/kg, (so US$2500/tonne), when this is added to the value of CO₂ abatement (e.g. US$50/tonne as a placeholder), and multiplied by 2.4 as noted previously, around US$2620/tonne of polycarbonate is available to work with. Hence, there is certainly some room to make an interesting case.
A quick review of "CO₂ – No Do" would conclude that it is worth continuing to explore the idea. Specific next steps would include a review of the technology and the cost, including power and water demand, plus exploring for a better understanding of the polycarbonate market relative to a potential pilot system.
Conclusion
Lastly, decarbonization that will make an impact is about doing difficult things and finding financially sensible solutions, which can scale and be replicated widely. Hopefully, the framework of questions presented here invokes some thought in either sifting through ideas or developing new technology.